我々の研究室では、イオン液体、電気化学、材料科学を基盤とした研究を行い、SDGsの達成に貢献できる様々なエネルギー材料、蓄電デバイス、資源循環プロセス、分析技術の開発に取り組んでいます。
Our laboratory conducts researches based on ionic liquids, electrochemistry, and materials science, and is working on the development of various energy materials, energy storage devices, resource recycling processes, and analytical techniques that can contribute to the achievement of the SDGs.
【イオンのみから構成される液体塩: イオン液体】
イオン液体は、液相温度域が低い、難揮発性、難燃性、イオン伝導性が比較的高いなどの特徴を併せ持つ特異な液体であるが、それらの特徴の中には、カチオン‒アニオン間の強くもなく、弱くもない、あいまいな強さの相互作用によって発現したものも少なくない。つまり、『イオン液体を分子レベルで理解することは、その物性発現の根源を知ること。』と言っても過言ではない。我々の研究室では、基礎科学に立脚したイオン液体の理解に取り組むとともに、イオン液体を機能性材料として使用する、反応場として利用するなど、多岐にわたるイオン液体の用途開発にチャレンジしています!
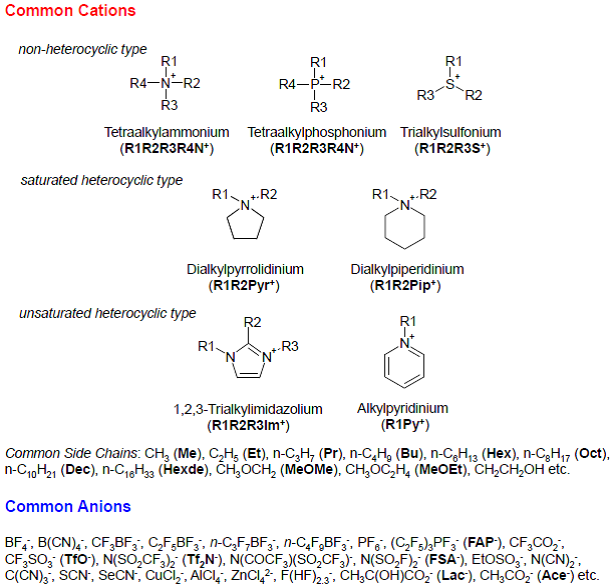
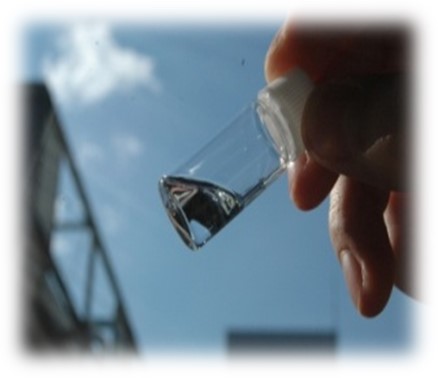
【Liquid salts composed only of ions: ionic liquids】
Ionic liquids (also called room-temperature molten salts or ambient-temperature molten salts) are a general term for salts that have a liquid phase at room temperature, i.e., those are composed only of cations (+ ions) and anions (- ions). In general, ionic species that constitute ionic liquids are organic ions and complex ions, and their volume is inevitably larger than that of monatomic ions. Therefore, the surface charge density of the ionic species forming the ionic liquid is lower than that of monatomic ions. In addition, since these ion species have various molecular vibrational modes, they have large entropy, and bulky ion species can be expected to reduce the interaction between ions due to the effect of steric hindrance. For these reasons, the melting points of ionic liquids are much lower than inorganic salts such as NaCl.
Ionic liquids are unique liquids that have a combination of features such as a low liquidus temperature range, low volatility, flame retardancy, and relatively high ionic conductivity. Some of these features are obtained by interactions between cations and anions that are neither strong nor weak. In other words, it is no exaggeration to say that “Understanding ionic liquids at the molecular level means knowing the origin of their physical properties. In our laboratory, we are working to understand ionic liquids based on basic science, and we are also trying to develop a wide range of applications for ionic liquids, such as using them as functional materials and reaction media!
【電気化学と材料科学】
電気化学は電子やイオンといった荷電粒子が関与する化学現象を対象とした学問であることから、対象となる領域は物理化学や無機化学、分析化学はもちろんのこと、何のかかわりもないように見える有機化学や生物化学にまで至る。そのため、電気化学反応は工業電解、電気めっき、電着、電池、センサー、半導体、生体内反応など多岐にわたって利用されている。一方、材料科学は物質固有の物性が発現する原因を明らかにし、その知見を利用して、新しい材料を創り出す学問である。電気化学と材料科学は相性が良く、エネルギー材料はこの2つの学問の境界領域にある研究対象と言える。エネルギー材料そのものを作製するだけでなく、エネルギー材料を利用して化学エネルギーを電気エネルギー(その逆もありうる)に変換するためのプロセスを分析・観察することもこの範疇に入る。
【Electrochemistry and Materials Science】
Since electrochemistry is a study that deals with chemical phenomena involving charged particles such as electrons and ions, the fields covered include not only physical chemistry, inorganic chemistry and analytical chemistry, but also organic chemistry and biochemistry. Therefore, electrochemical reactions are widely used in industrial electrolysis, electroplating, electrophoretic coating, batteries, sensors, semiconductors, and in vivo reactions. Materials science, on the other hand, is a field of study that clarifies the causes of the manifestation of inherent physical properties in substances, and uses that knowledge to create new materials. Electrochemistry and materials science go well together, and energy materials can be said to be a research subject at the boundary between these two disciplines. This includes not only synthesizing the energetic materials themselves, but also analyzing and observing the processes by which the energetic materials are used to convert chemical energy into electrical energy (and vice versa).
【イオン液体 × 電気化学 × 材料科学 + 低環境負荷 + 汎用元素 = ? 】
我々の研究室では、イオン液体、電気化学、材料科学の3つに、SDGsを達成するために欠かせない2つのキーワードである低環境負荷と汎用元素を加えることで、人類の未来を支える材料やデバイス、リサイクル技術を創造することを目指しています。それを実現するため、津田がこれまでに行ってきた研究の例を紹介します。
【Ionic liquids × electrochemistry × materials science + low environmental impact + common elements = ? 】
In our laboratory, we aim to create novel materials, devices, and recycling technologies that support the future of mankind, by adding two keywords, low environmental impact and general-purpose elements, which are indispensable for achieving the SDGs, to the three research fields of ionic liquids, electrochemistry, and materials science. In order to realize this, Here are some examples of researches that Tsuda has conducted.
【新しいイオン液体の開発】
合成化学的手法によって有機カチオンの分子設計を行う、錯イオンの構造を制御するなどの手法を駆使して、ターゲットとする物性をイオン液体に発現させることができる。例えば、後者については、アニオン種の構造にチオシアン酸基などの官能基を導入することでエントロピーの増大を誘起したり、アニオンの構造対称性を低下させることにより、液相温度域の低下と電気化学反応において極めて重要なパラメーターである輸送物性の改善することに成功している。このようなアプローチによって、有機塩を使用することなく、無機塩のみでイオン液体(無機イオン液体)を調製することが可能になった。
Development of Novel Ionic Liquids
By making full use of methods such as molecular design of organic cations using synthetic chemical methods and controlling the structure of complex ions, it is possible to gain target physical properties in ionic liquids. For the latter, for example, introducing a functional group such as a thiocyanate group into the structure of the anion species induces an increase in entropy and decrease in the structural symmetry of the anion. As a result, we have succeeded in lowering the liquidus temperature range and the electrical improving transport properties of ionic liquids, which are extremely important parameters in electrochemical reactions. Such an approach has made it possible to prepare ionic liquids using only inorganic salts without using organic salts (inorganic ionic liquids).
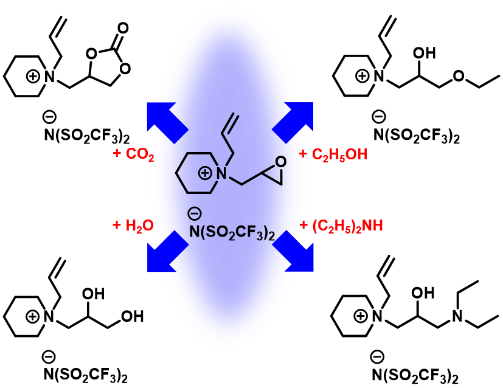
エネルギー材料のワンポット調製
我々の社会が様々な材料によって支えられていることはよく知られているが、その中には直接的に目にすることの少ない微小なサイズの材料もある。その代表例がナノ粒子である。材料のナノ粒子化は質量あたりの比表面積を増大させるだけでなく、量子サイズ効果の発現に寄与することも少なくない。しかし、ナノ粒子は表面エネルギーが高く、容易に凝集するため、安定化剤(アミン、チオール、高分子など)による表面修飾が必要不可欠である。しかし、興味深いことに、イオン液体をナノ粒子の調製用反応媒体として用いた場合、安定化剤を使用することなく、調製したナノ粒子はイオン液体によって安定化される。ただし、安定化剤ほどの強い相互作用はないようである。我々はこの知見を利用して、イオン液体中にターゲットとする金属種を含む前駆体(例えば、Ptであれば、白金アセチルアセトナト(Pt(acac)2)など)を加え、溶解または単分散させたのち、加熱するだけでターゲットとする金属や合金のナノ粒子を得ることができることを見い出した【イオン液体-熱分解法】 (Fig. 1)。本手法を行う際に、ナノ粒子を担持させたい炭素材料を一緒に混ぜておくと、ワンポットプロセスでナノ粒子が担持された炭素材料を作ることができ、エネルギー材料として評価を行っている。このプロセスはスケールアップが容易であるため、工業化が容易であるといった特徴もある。
One-Pot Preparation of Energy Materials
It is well known that our modern life is supported by various materials, but some of them are microscopic materials that we rarely see directly. A typical example is nanoparticles. Making bulk materials into nanoparticles not only increases the specific surface area per mass, but also often develops the quantum size effect. However, since nanoparticles have high surface energy and easily aggregate, surface modification with stabilizers (amines, thiols, polymers, etc.) is essential. Interestingly, however, when an ionic liquid is used as the reaction medium for the preparation of nanoparticles, the resulting nanoparticles are stabilized by the ionic liquid without the use of a stabilizing agent. The interaction appears to be weaker than with stabilizers. Using this knowledge, we found a new approach for nanoparticle preparation [ionic liquid-pyrolytic method] consisting of a process of adding a precursor containing the target metal species (e.g., platinum(II) acetylacetonate (Pt(acac)2) for Pt) to ionic liquid and a process of heating the ionic liquid with the precursor. When using this method, if the carbon materials to be supported with nanoparticles are mixed together, a nanoparticle-supported carbon material can be produced in a one-pot process, and is being evaluated as an energy material. Since this process is easy to scale up, it also has the feature of being easy to industrialize.
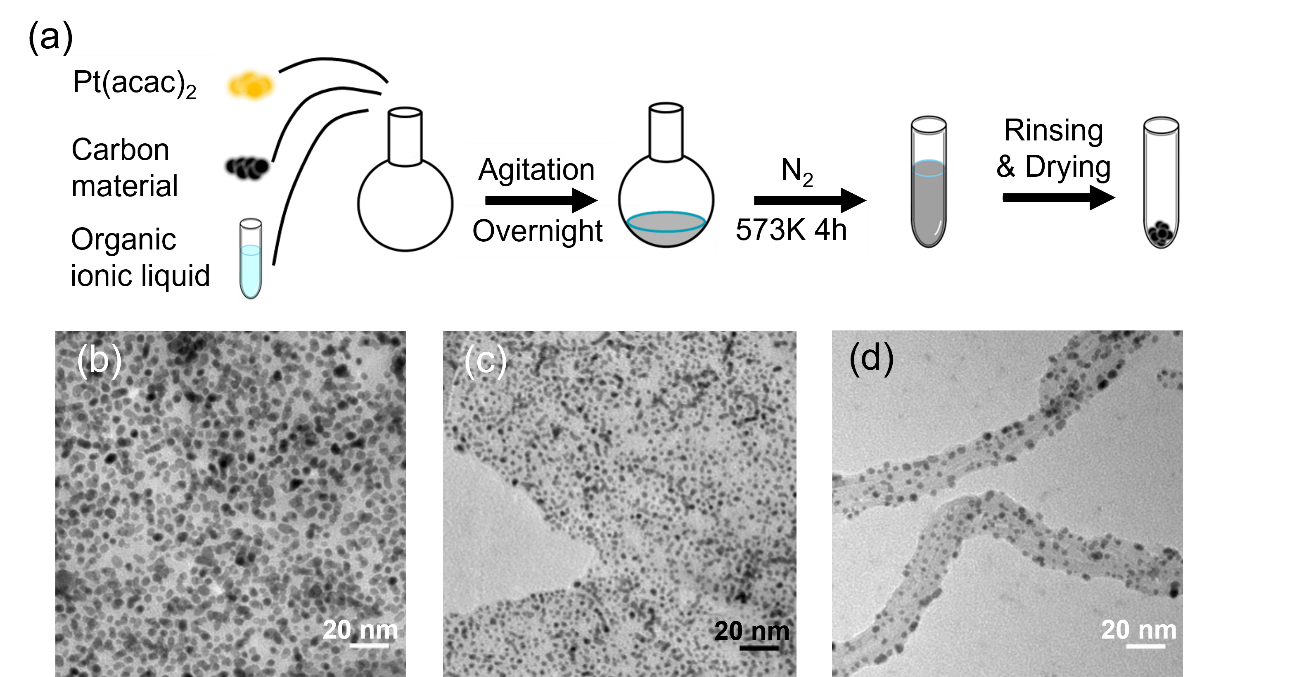
Fig. 1 (a) Outline of a Pt nanoparticle supported carbon material preparation process by IL-pyrolysis method. (b-d) TEM images of Pt nanoparticle supported carbon materials prepared in [C4mim][Tf2N]. The carbon materials were (b) thick graphene nanoplatelets (20-30 graphene layers, GNP-20), (c) thin graphene nanoplatelets (< 3 graphene layers, GNP-3), and (d) MWCNT.
電気化学反応のその場(in situ, operando)観察
二次電池はユビキタス社会や低炭素化社会を支える重要な蓄電デバイスであり、その適用範囲は広がる一方である。それに伴い、より高性能な二次電池の登場が期待されている。特に、電池の高容量化については自動車業界からのニーズが高く、熾烈な研究開発競争が世界的に繰り広げられている。このような背景から、より多くの電気を化学エネルギーとして貯め込むことのできる電極活物質に関する報告が数多く存在し、既存のリチウムイオン電池用活物質の容量を大きく超えるものも珍しくない。しかしながら、充放電容量の飛躍的な増大は活物質の著しい体積変化を引き起こし、電池の劣化を加速する。そのため、充放電時における活物質の形態変化を正しく理解することは、電池性能の向上に直結する極めて重要な情報となる。
イオン液体は難揮発性、非帯電性、イオン伝導性を有する室温で液体の塩であり、我々はこれを利用した電子顕微鏡観察法の構築に取り組んできた。この観察法の特徴は電解質が液体であるにもかかわらず、密閉セルを使用することなく像が得られる点にあり、電気化学反応の観察時においても例外ではない。イオン液体電解液を用いて電池反応をその場観察する際のセルやホルダーの例をFig. 2に示している。我々はこれまでに、シリコン、リチウム、マグネシウム、亜鉛といった次世代二次電池用高容量負極活物質のイオン液体電解液中における充放電挙動をその場SEM/TEM観察によって調査することに成功しており、その知見を負極活物質の材料設計にフィードバックしている。ここで蓄積したその場SEM/TEM観察のノウハウは分光分析などへ展開することも可能である。
In situ / Operando Observation of Electrochemical Reactions
Secondary batteries are important power storage devices that support a ubiquitous society and a low-carbon society, and their scope of application continues to expand. Along with this, the advent of secondary batteries with higher performance is expected. In particular, there is a high demand from the automobile industry for high-capacity batteries, and fierce research and development competition is taking place worldwide. Against this background, there are many reports on electrode active materials that can store more electricity as chemical energy, and it is not uncommon for the capacity to greatly exceed the capacity of existing active materials for lithium ion batteries. However, a dramatic increase in charge/discharge capacity causes a significant change in volume of the active material, accelerating deterioration of the battery. Therefore, correct understanding of the morphological change of the active material during charging and discharging is extremely important information that directly leads to the improvement of battery performance.
Ionic liquids have negligible vapor pressure, flame-retardant, and anti-static nature. Using this feature, we have been working on the electron microscope observation techniques. The feature of this observation method is that an image can be obtained without using a closed cell even though the electrolyte is liquid, and the observation of electrochemical reactions is no exception. Figure 2 shows examples of cells and a holder for operando/in situ electron microscope observation of battery reactions using an ionic liquid electrolyte. We have so far succeeded in investigating the charge-discharge behavior of high-capacity negative electrode active materials for next-generation secondary batteries such as silicon, lithium, magnesium, and zinc in ionic liquid electrolytes by in-situ SEM/TEM observation. This knowledge is fed back to the material design of the negative electrode active material. Know-how accumulated here concerning the in-situ SEM/TEM observation can also be applied to spectroscopic analysis.
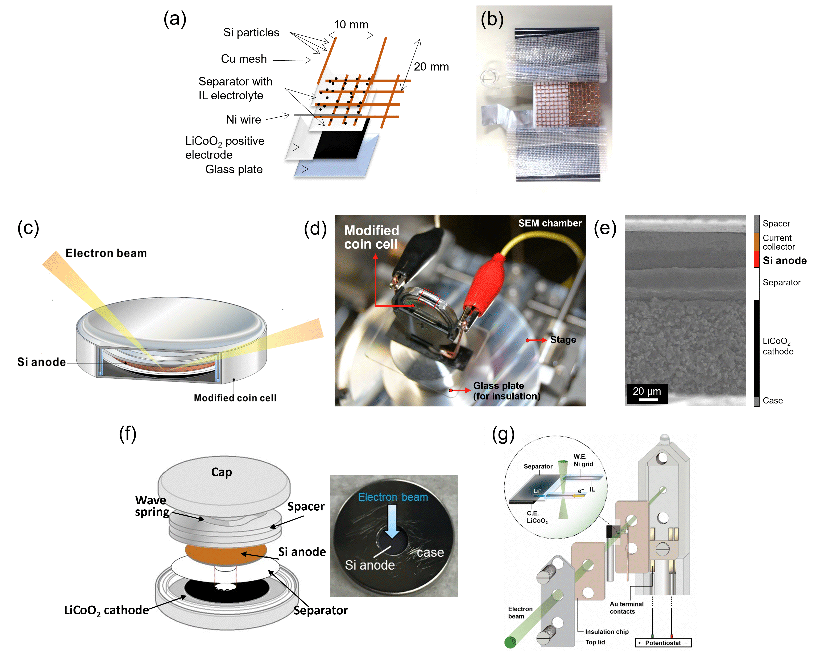
Fig. 2 Schematic drawings and photographs of (a, b) the three-electrode cell, (c, d, f) the coin-type cell for in situ/operando SEM observation and (g) the window-less miniature cell for in situ TEM observation. (e) SEM image of a cross-sectional view of the coin-type cell depicted in Fig. 2d. (f) This coin-type cell is used for obtaining the information on the variation in anode surface during battery reactions.
アルミニウムハイアップグレードリサイクル
世界人口の爆発的な増加は資源使用量の増大を引き起こし、鉄やアルミニウムといった地殻存在量の多い金属でさえ、リサイクルなしでは将来的に枯渇する可能性が高い。また、それら金属の製錬プロセスによって大量の温室効果ガスが放出される。金属の水平リサイクルプロセスは資源の有効利用と新地金の製造に伴う環境負荷の低減を可能とすることから、ますますその重要性は高まると考えられる。このような背景から、最近では塩化アルミニウム(AlCl3)系イオン液体を用いたアルミニウム電気めっき技術の水平リサイクルプロセスへの展開が検討されている。アルミニウムはホール・エルー法による生産に必要な電力原単位が13,000 ~ 15,000 kWh t-1にもなることから、【アルミニウムは電気の缶詰】と揶揄されることも多いが、リサイクルに必要なエネルギー量は新地金の製錬時に投入されるエネルギー量のわずか3 ~ 5 %程度である。しかし、このリサイクルによって得られるAlの純度は新地金に劣る。つまり、既存のプロセスでの水平リサイクルは困難と言える。リサイクルアルミニウムにはシリコンを中心とした不純物が蓄積し、最終的にはスクラップアルミニウムとして廃棄されることになる。今後、その廃棄量は増加の一途を辿ると予想されている。我々は、塩化アルミニウム系イオン液体を用いることで、スクラップアルミニウムを低電力原単位で新地金のレベルにまで高純度化できる工業プロセスの開発に取り組んでいる。
Aluminum High Upgrade Recycling
Explosive growth in the world’s population will lead to increased resource use, and even metals with high abundance in the earth’s crust, such as iron and aluminum, are likely to be depleted in the future without recycling. Also, the smelting process of these metals releases large amounts of greenhouse gases. The horizontal metal recycling process is expected to become more and more important because it enables effective utilization of resources and reduction of the environmental burden associated with the production of new ingots. In this context, recently, the application of aluminum electroplating technology using aluminum chloride (AlCl3)-based ionic liquid to a horizontal recycling process has been investigated. Since aluminum requires 13,000 to 15,000 kWh t-1 of electric power for production by the Hall-Heroult method, it is often ridiculed that aluminum is canned electricity. But, the amount of energy required for recycling is only about 3 to 5% of the amount of energy input during the smelting of new ingots. Unfortunately, the purity of Al obtained by this recycling is inferior to virgin metal. In other words, it can be said that horizontal recycling in the existing process is difficult. Repeatedly recycled aluminum accumulates impurities, mainly silicon, and is eventually discarded as scrap aluminum. It is expected that the amount of waste will continue to increase in the future. We are working on the development of an industrial process that can purify scrap aluminum to the level of new ingots with low power consumption by using AlCl3-based ionic liquids.
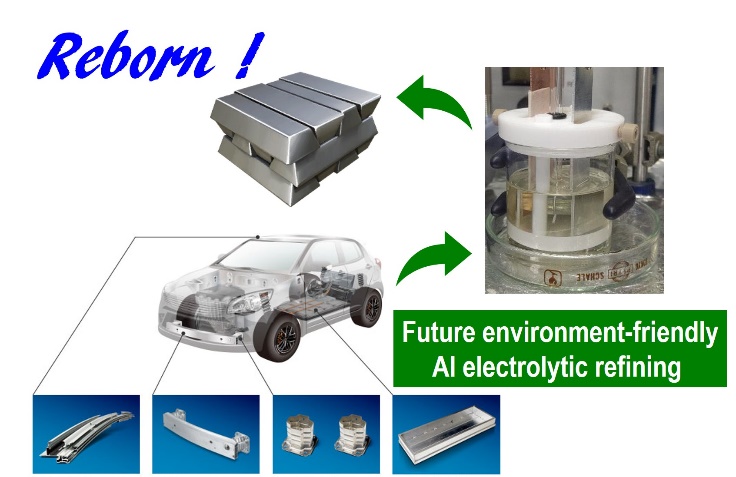
アルミニウム金属負極アニオン二次電池
ユビキタス社会、低炭素化社会への急速な移行によって蓄電池に対する期待は高まり続けており、その適用範囲は拡大し続けている。しかし、蓄電池を構成する元素のなかには、資源量に不安を抱えるものもあり、これまでのように単に電池性能だけを追い求めるのではなく、資源量といった観点から蓄電池を設計し、創ることも重要になってきている。例えば、リチウムイオン電池に取って代わる蓄電池として、リチウムイオンと同じアルカリ金属イオンであるナトリウムイオンやカリウムイオンを用いた電池系が提案され、代替電池としての利用が実現しつつある。最近では、マグネシウム(3833 mAh cm−3, 2205 mAh g−1)や亜鉛(5854 mAh cm−3, 820 mAh g−1)、アルミニウム(8046 mAh cm−3, 2980 mAh g−1)のような高容量汎用多価金属を負極に用いた電池系に関する研究も活発化している(括弧内の値は理論体積容量(mAh cm−3)と理論重量容量(mAh g−1)を示しており、 リチウムは、2066 mAh cm−3、3862 mAh g−1である。)。理論体積容量で比較すると、上述した3つの多価金属の方がリチウムよりも大きい。限られたスペースで電池を配置しなければいけない場合、これらの金属負極を使った電池が有利になることも十分に想定される。
電池を構成する主要部材は負極、正極、電解質である。これらには多種多様な元素が利用されており、汎用元素だけで蓄電池系を構築することは意外に難しい。我々は資源量が豊富であり、極めて良好なクーロン効率や高容量が期待できるアルミニウム金属負極を利用したアニオン二次電池に関する研究を行っている(Fig. 3)。電池材料として魅力的なアルミニウム金属負極を用いる点に、この電池系の特徴があるのはもちろんのこと、汎用元素のみで構成された電池主要部材が利用できるメリットも大きい。低炭素化社会を達成するには、自然エネルギーを使って得られた電気エネルギーをシームレスに使用するための電力平準化用蓄電池システムの導入が必須である。Al金属負極アニオン電池は汎用元素だけで作製でき、大量生産に対する障壁が小さいため、その重要な役割を担う可能性を秘めている。
Aluminum Metal Anode Anion-Secondary Battery
Due to the rapid transition to a ubiquitous society and a low-carbon society, expectations for storage batteries continue to rise, and the scope of their application continues to expand. However, some of the elements that make up secondary batteries have concerns about the amount of resources available, so it is important to design and create secondary batteries from the perspective of resource, instead of simply pursuing battery performance as in the past. For example, as secondary batteries to replace lithium-ion batteries, battery systems using sodium ions and potassium ions, which are alkali metal ions similar to lithium ions, have been proposed and are being used as alternative batteries. Recent years, research on battery systems using high-capacity common polyvalent metals, e.g., magnesium (3833 mAh cm-3, 2205 mAh g-1), zinc (5854 mAh cm-3, 820 mAh g-1) and aluminum (8046 mAh cm-3, 2980 mAh g-1), for the anode is also active (Values in parentheses indicate theoretical volume capacity (mAh cm-3) and theoretical weight capacity (mAh g-1). Cf. Lithium is 2066 mAh cm-3, 3862 mAh g-1.). Comparing the theoretical volume capacities, the three polyvalent metals mentioned above are larger than lithium. It is fully assumed that batteries using these metal negative electrodes will be advantageous when batteries must be arranged in a limited space.
The main components that make up a battery are an anode, a cathode, and an electrolyte. A wide variety of elements are used for these, and it is unexpectedly difficult to construct a secondary battery system using only general-purpose elements. We are conducting research on anion secondary batteries using aluminum metal anodes, which are abundant resources and are expected to have extremely good coulombic efficiency and high capacity (Fig. 3). The most distinctive feature of this battery system is the use of an attractive aluminum metal negative electrode as a battery material. Besides, it should not be forgotten that the main components of the battery can be made only of common elements. In order to achieve a low-carbon society, it is essential to introduce a secondary battery system for power leveling that enables continuous use of electrical energy obtained using natural energy. Since Al metal anode anion-secondary batteries can be fabricated using only general-purpose elements and the barriers to mass production are small, they have the potential to play an important role.
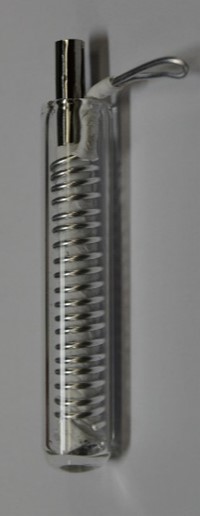
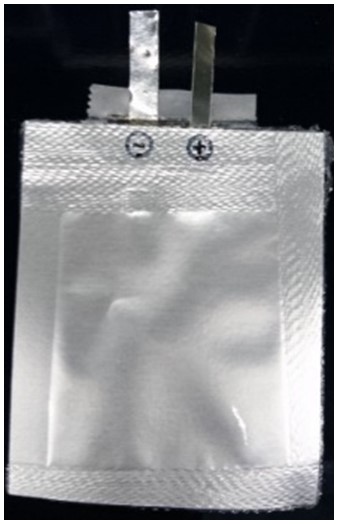
Fig. 3 Typical examples of Al metal anode anion-secondary battery.